Student researchers expand neutrino knowledge
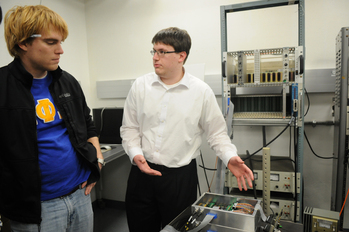
By Claire Deahl | The Pitt News
[The Pitt News, Nov. 20, 2012, pg. 1 print edition]
As you reach the end of this clause, trillions of research subjects have zipped through your body. They’re named, in Italian, “little neutral ones.” They travel at close to the speed of light, and they may unlock the secrets of the universe — or so hopes a team of Pitt student researchers.
Under the guidance of Vittorio Paolone, associate professor in the Department of Physics and Astronomy, three Pitt students are getting to know the “neutrino,” a tiny, uncharged and poorly understood member of the soup of elementary particles that composes our known world.
Using computer simulations, juniors Bruce Howard and Tom Carroll and post-baccalaureate student Maher Elharake are laying conceptual groundwork for a large experiment in Europe that could shed light on neutrinos and, perhaps, how we came to be.
“It could potentially explain why things are the way they are,” Howard said.
A strange existence
The study of neutrinos could solve a long-standing conundrum among physicists: Isaac Newton’s apple. Of course, the cause of the apple’s proverbial descent onto Newton’s head is settled. What isn’t is the fact that his apple existed in the first place.
As odd as it might seem, the everyday matter contained in apples and zebrafish doesn’t fit snugly in the standard model of physics. That is, accepted physics would predict that conditions of the early universe should have destroyed matter, leaving nothing but a sea of radiation today.
Under the standard model, the Big Bang should have produced equal amounts of matter and its counterpart, antimatter, both of which would annihilate each other on the spot.
But a mere apple in the hand (or on the head) indicates that the standard model could use some revision. Despite the fact that matter and antimatter annihilate in physics experiments, matter dominates in our universe, so “something must prefer matter over antimatter,” Paolone said.
“People think it’s got to do with neutrinos,” Paolone added.
The particle physics world has arrived at neutrinos after an elimination process of sorts. The idea is that some particle must exhibit a “charge-parity violation” — which would mean the particle behaves differently than its antiparticle — big enough to account for matter’s early victory over antimatter.
To date, physicists have not found a sufficiently large CP violation in any observable particle, discovering only a small amount in a specific type of “quark” — another fundamental particle.
“Something had to have done it. So the next thing is to go to neutrinos,” Paolone said.
But the attention paid to neutrinos lies in more than just faith in a remaining alternative. Rather, “It turns out that neutrinos oscillate,” said Howard. “One neutrino, which classically you’d like to think of as a point-like particle, is a superposition of three different mass states.”
He means that neutrinos, as they travel through space, interconvert between mass states, which are mixtures of mass contributions of three different “flavors” of neutrinos: the electron, muon and tau neutrinos.
Scientists hope that if they can better observe neutrinos’ oscillation from state to state and from flavor to flavor, they might discover a sufficient CP violation in the oscillation process.
“If CP violation doesn’t exist in neutrinos, then forget it because we can’t explain it,” Paolone said. “But it’s expected to be very large with neutrinos.”
Zeroing in on neutrinos
Only experiments will tell if neutrino oscillation exhibits CP violation, and if so, by how much. Howard, Carroll and Elharake understand this.
“Because we’re experimentalists, we want to test it,” Carroll said.
However, testing it directly will require brain and capital resources far beyond the capacities of any individual laboratory such as Paolone’s. Particle physics sets itself apart from other fields in that asking an experimental question typically means a lot of money and collaboration between many scientists and universities: The well-known Large Hadron Collider involved thousands of professors.
Finding answers to the next big questions regarding neutrino behavior will take a similarly collaborative route when a large, multi-location particle detector begins studying neutrinos at the European Center for Nuclear Research in Geneva in 2014.
Paolone’s students have grabbed on to a piece of that puzzle: They’re running simulations of the CERN detector with hopes of enhancing its detection capacity but, interestingly, not of neutrinos.
“The experiments that we’re a part of want to observe these neutrino oscillations from a man-made beam of neutrinos,” Carroll said. “We’re trying to understand the beginning [of that beam],” he said.
But physicists can’t produce neutrinos out of thin air. They first have to produce — you guessed it — a different class of particles.
Enter the “hadrons.” The CERN detector, which replicates a detector at Fermilab near Chicago, is really three parts: a particle smasher, magnets to aim the resulting particles and detectors to monitor what’s happened.
First, a certain type of hadron (the proton) is shot at a block of carbon, and the collision kicks off a shower of other hadrons. Large magnets line up the emitted hadrons, and the hadrons then follow a predictable decay process that yields neutrinos.
The neutrinos travel in a beam, passing through solid Earth and oscillating between mass states to detectors miles away.
The problem is that no one knows for sure which hadrons decayed into which neutrinos, and without such certainty, say goodbye to discovering CP violation in neutrinos — to get a sense of why, try precisely diagnosing a patient without taking a medical history. Boosting certainty is where CERN and Paolone’s students come in.
“What happens the moment we start producing these particles, when we take this proton and we smash it into carbon?” Carroll said.
“The goal of [the CERN experiment] is to better constrain the production of hadrons that come out of the block,” Howard said, and he and his fellow students are helping educate those “constraints.”
Contrary to past neutrino experiments, the CERN detector will have the ability to measure the hadrons emitted — beforehand, however, the scientists will need to know where, and where not, to look. To that end, the work of Howard, Carroll and Elharake will help.
Students and their particles
Since this past summer, the student team has tried to characterize the emitted hadrons by simulating the CERN detector with computer programs. Their results could tell scientists how to calibrate the CERN detector so as to best observe the hadrons that shower from the block.
“In the simulations we have all the physics we know,” Carroll said. “When we start collecting actual experimental data at [CERN], we’re going to use our simulations to dictate where it looks like where we want to collect data.”
Such a clearer picture of emitted hadrons will allow CERN experimentalists to correlate the hadrons’ characteristics with those of the hadrons’ decay products — neutrinos. That relationship could then elucidate neutrino oscillations wherein CP violation — i.e., a key to the universe’s secrets — might reside.
In chasing explanations for the physical world, the Pitt team has found no light exercise. The labors of creating computer programs for simulations have dominated the past few months for Howard, Carroll and Elharake.
“They’re awful,” Elharake said, referring to pesky errors and problems that frustrate the computer programmer. “It’s not my greatest interest, to put it kind of bluntly.”
But the students have developed strategies to address these frustrations.
Elharake makes use of his surrounding human resources. “I had a lot of questions for Bruce and Tom, and they were most generous in their being able to help me through pretty much all of [the programming issues],” he said.
Carroll applies brute force. “If I hit a wall, I like to power through it,” he said. “I try not to go to sleep without a major problem solved.”
Howard prefers to relax and regroup. “Sometimes you just have to take a break for a few minutes and then get a drink of water and come back and be mentally prepared to read through several lines of code to see where that one error is,” he said.
The difficulties help make the undergraduate research experience worthwhile, according to Paolone. “You learn a lot more from doing things, from banging your head against the wall a few times,” he said. “[My students] have to learn how to get frustrated and then figure out how to solve the problem.”
The students justify their efforts on other grounds.
Howard emphasizes how the experience has solidified his intention to pursue particle physics beyond Pitt and how it has included him in a rewarding collaboration.
“It’s a way to begin that intense period of learning to where I can actually continue to keep doing this research for the rest of my life,” he said. “Also it’s pretty cool that the stuff that we’re doing is helping other experiments on neutrinos as well.”
Elharake, who intends to be a physician, thinks particle research adds something valuable to his education that he does not expect to receive from medical training. “It helps me get insight into things I won’t have the chance to do much from here on out,” he said. “It’s more to expand my horizons.”
And though probing the universe’s most fundamental mysteries invigorates each student, this is especially the case for Carroll, who, like Howard, looks forward to physics graduate school. “Wanting to know the answer, wanting to come up with the solution to the problem is what drives me,” he said. “That’s why I’ll stay up late programming.”
As you reach the end of this clause, trillions of research subjects have zipped through your body. They’re named, in Italian, “little neutral ones.” They travel at close to the speed of light, and they may unlock the secrets of the universe — or so hopes a team of Pitt student researchers.
Under the guidance of Vittorio Paolone, associate professor in the Department of Physics and Astronomy, three Pitt students are getting to know the “neutrino,” a tiny, uncharged and poorly understood member of the soup of elementary particles that composes our known world.
Using computer simulations, juniors Bruce Howard and Tom Carroll and post-baccalaureate student Maher Elharake are laying conceptual groundwork for a large experiment in Europe that could shed light on neutrinos and, perhaps, how we came to be.
“It could potentially explain why things are the way they are,” Howard said.
A strange existence
The study of neutrinos could solve a long-standing conundrum among physicists: Isaac Newton’s apple. Of course, the cause of the apple’s proverbial descent onto Newton’s head is settled. What isn’t is the fact that his apple existed in the first place.
As odd as it might seem, the everyday matter contained in apples and zebrafish doesn’t fit snugly in the standard model of physics. That is, accepted physics would predict that conditions of the early universe should have destroyed matter, leaving nothing but a sea of radiation today.
Under the standard model, the Big Bang should have produced equal amounts of matter and its counterpart, antimatter, both of which would annihilate each other on the spot.
But a mere apple in the hand (or on the head) indicates that the standard model could use some revision. Despite the fact that matter and antimatter annihilate in physics experiments, matter dominates in our universe, so “something must prefer matter over antimatter,” Paolone said.
“People think it’s got to do with neutrinos,” Paolone added.
The particle physics world has arrived at neutrinos after an elimination process of sorts. The idea is that some particle must exhibit a “charge-parity violation” — which would mean the particle behaves differently than its antiparticle — big enough to account for matter’s early victory over antimatter.
To date, physicists have not found a sufficiently large CP violation in any observable particle, discovering only a small amount in a specific type of “quark” — another fundamental particle.
“Something had to have done it. So the next thing is to go to neutrinos,” Paolone said.
But the attention paid to neutrinos lies in more than just faith in a remaining alternative. Rather, “It turns out that neutrinos oscillate,” said Howard. “One neutrino, which classically you’d like to think of as a point-like particle, is a superposition of three different mass states.”
He means that neutrinos, as they travel through space, interconvert between mass states, which are mixtures of mass contributions of three different “flavors” of neutrinos: the electron, muon and tau neutrinos.
Scientists hope that if they can better observe neutrinos’ oscillation from state to state and from flavor to flavor, they might discover a sufficient CP violation in the oscillation process.
“If CP violation doesn’t exist in neutrinos, then forget it because we can’t explain it,” Paolone said. “But it’s expected to be very large with neutrinos.”
Zeroing in on neutrinos
Only experiments will tell if neutrino oscillation exhibits CP violation, and if so, by how much. Howard, Carroll and Elharake understand this.
“Because we’re experimentalists, we want to test it,” Carroll said.
However, testing it directly will require brain and capital resources far beyond the capacities of any individual laboratory such as Paolone’s. Particle physics sets itself apart from other fields in that asking an experimental question typically means a lot of money and collaboration between many scientists and universities: The well-known Large Hadron Collider involved thousands of professors.
Finding answers to the next big questions regarding neutrino behavior will take a similarly collaborative route when a large, multi-location particle detector begins studying neutrinos at the European Center for Nuclear Research in Geneva in 2014.
Paolone’s students have grabbed on to a piece of that puzzle: They’re running simulations of the CERN detector with hopes of enhancing its detection capacity but, interestingly, not of neutrinos.
“The experiments that we’re a part of want to observe these neutrino oscillations from a man-made beam of neutrinos,” Carroll said. “We’re trying to understand the beginning [of that beam],” he said.
But physicists can’t produce neutrinos out of thin air. They first have to produce — you guessed it — a different class of particles.
Enter the “hadrons.” The CERN detector, which replicates a detector at Fermilab near Chicago, is really three parts: a particle smasher, magnets to aim the resulting particles and detectors to monitor what’s happened.
First, a certain type of hadron (the proton) is shot at a block of carbon, and the collision kicks off a shower of other hadrons. Large magnets line up the emitted hadrons, and the hadrons then follow a predictable decay process that yields neutrinos.
The neutrinos travel in a beam, passing through solid Earth and oscillating between mass states to detectors miles away.
The problem is that no one knows for sure which hadrons decayed into which neutrinos, and without such certainty, say goodbye to discovering CP violation in neutrinos — to get a sense of why, try precisely diagnosing a patient without taking a medical history. Boosting certainty is where CERN and Paolone’s students come in.
“What happens the moment we start producing these particles, when we take this proton and we smash it into carbon?” Carroll said.
“The goal of [the CERN experiment] is to better constrain the production of hadrons that come out of the block,” Howard said, and he and his fellow students are helping educate those “constraints.”
Contrary to past neutrino experiments, the CERN detector will have the ability to measure the hadrons emitted — beforehand, however, the scientists will need to know where, and where not, to look. To that end, the work of Howard, Carroll and Elharake will help.
Students and their particles
Since this past summer, the student team has tried to characterize the emitted hadrons by simulating the CERN detector with computer programs. Their results could tell scientists how to calibrate the CERN detector so as to best observe the hadrons that shower from the block.
“In the simulations we have all the physics we know,” Carroll said. “When we start collecting actual experimental data at [CERN], we’re going to use our simulations to dictate where it looks like where we want to collect data.”
Such a clearer picture of emitted hadrons will allow CERN experimentalists to correlate the hadrons’ characteristics with those of the hadrons’ decay products — neutrinos. That relationship could then elucidate neutrino oscillations wherein CP violation — i.e., a key to the universe’s secrets — might reside.
In chasing explanations for the physical world, the Pitt team has found no light exercise. The labors of creating computer programs for simulations have dominated the past few months for Howard, Carroll and Elharake.
“They’re awful,” Elharake said, referring to pesky errors and problems that frustrate the computer programmer. “It’s not my greatest interest, to put it kind of bluntly.”
But the students have developed strategies to address these frustrations.
Elharake makes use of his surrounding human resources. “I had a lot of questions for Bruce and Tom, and they were most generous in their being able to help me through pretty much all of [the programming issues],” he said.
Carroll applies brute force. “If I hit a wall, I like to power through it,” he said. “I try not to go to sleep without a major problem solved.”
Howard prefers to relax and regroup. “Sometimes you just have to take a break for a few minutes and then get a drink of water and come back and be mentally prepared to read through several lines of code to see where that one error is,” he said.
The difficulties help make the undergraduate research experience worthwhile, according to Paolone. “You learn a lot more from doing things, from banging your head against the wall a few times,” he said. “[My students] have to learn how to get frustrated and then figure out how to solve the problem.”
The students justify their efforts on other grounds.
Howard emphasizes how the experience has solidified his intention to pursue particle physics beyond Pitt and how it has included him in a rewarding collaboration.
“It’s a way to begin that intense period of learning to where I can actually continue to keep doing this research for the rest of my life,” he said. “Also it’s pretty cool that the stuff that we’re doing is helping other experiments on neutrinos as well.”
Elharake, who intends to be a physician, thinks particle research adds something valuable to his education that he does not expect to receive from medical training. “It helps me get insight into things I won’t have the chance to do much from here on out,” he said. “It’s more to expand my horizons.”
And though probing the universe’s most fundamental mysteries invigorates each student, this is especially the case for Carroll, who, like Howard, looks forward to physics graduate school. “Wanting to know the answer, wanting to come up with the solution to the problem is what drives me,” he said. “That’s why I’ll stay up late programming.”